Custom Optical System Development
Add optics expertise to your team.

Great Design and Fast Delivery
“I was very happy with this design and system. It
was more than I expected and it works very well.
Great prototype and delivery was fast.”
Todd Lizotte, Hitachi Via Mechanics
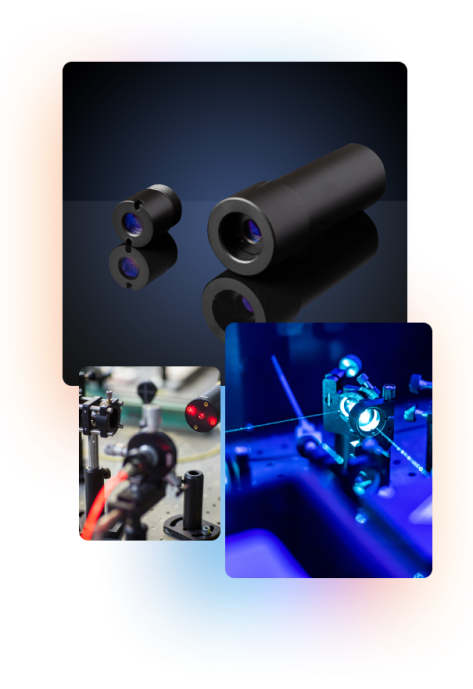
Precision Optical Engineering and Manufacturing Solutions
Custom solutions for your optical needs, from design to production.
Trusted By Industry Leaders Worldwide

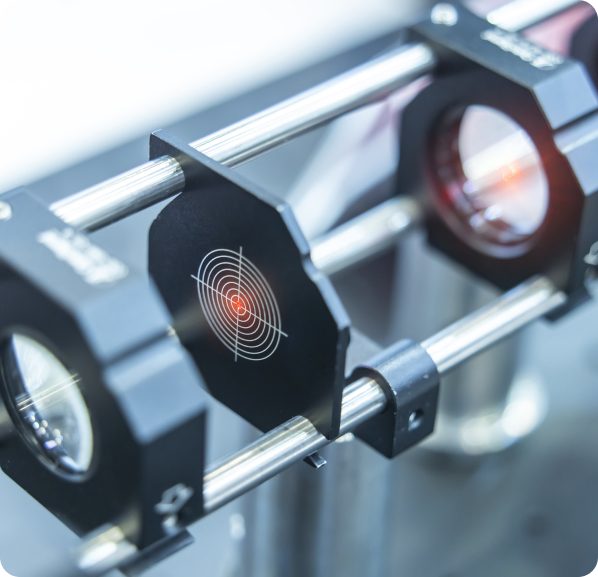
Leading the Way in Optical Engineering
At Optics for Hire, we pride ourselves on our unparalleled expertise in optical engineering. Our innovative solutions and precision in optical design and custom lens manufacturing set us apart. With a history of successful projects and a commitment to excellence, we ensure your project’s success with cutting-edge technology and efficient delivery.
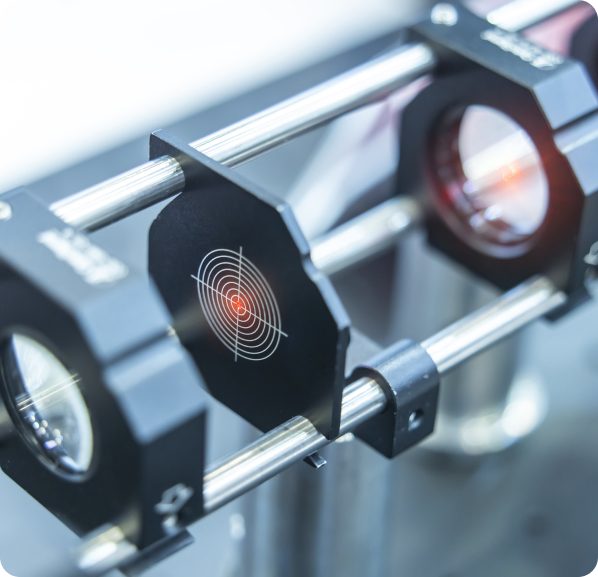
Leading the Way in Optical Engineering
At Optics for Hire, we pride ourselves on our unparalleled expertise in optical engineering. Our innovative solutions and precision in optical design and custom lens manufacturing set us apart. With a history of successful projects and a commitment to excellence, we ensure your project’s success with cutting-edge technology and efficient delivery.
How We Can Help
We assist clients in every stage of optical system development, including optical modeling, custom lens design, complete opto-electronic systems, applied optics research, and prototyping to volume manufacturing.
Optical Engineering & Custom Lens Design
Optical Engineering & Custom Lens Design
Opto
Electronics
Opto
Electronics
Optical Modeling
& Ray Tracing
Optical Modeling
& Ray Tracing
Illumination & Led
Optic Design
Illumination & Led
Optic Design
Prototyping to Volume
Manufacturing
Prototyping to Volume
Manufacturing
Complete Opto-Electronic Opto-Mechanical Systems
Complete Opto-Electronic Opto-Mechanical Systems
Applied Optics Research
& Consulting
Applied Optics Research
& Consulting
Lens Testing
Lens Testing
World Changing Collaborations
Groundbreaking optical technology we’ve helped launch.
Velodyne
Design of first commercial LIDAR lenses enables launch of Waymo/ Google self driving cars
2006
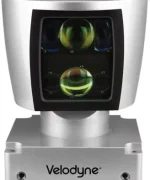
PrimeSense ( Sold to Apple)
Design of imaging lens for High-Energy X-ray Microscope to non-destructively study material stress in areas from 1cm square to atomic level
2009
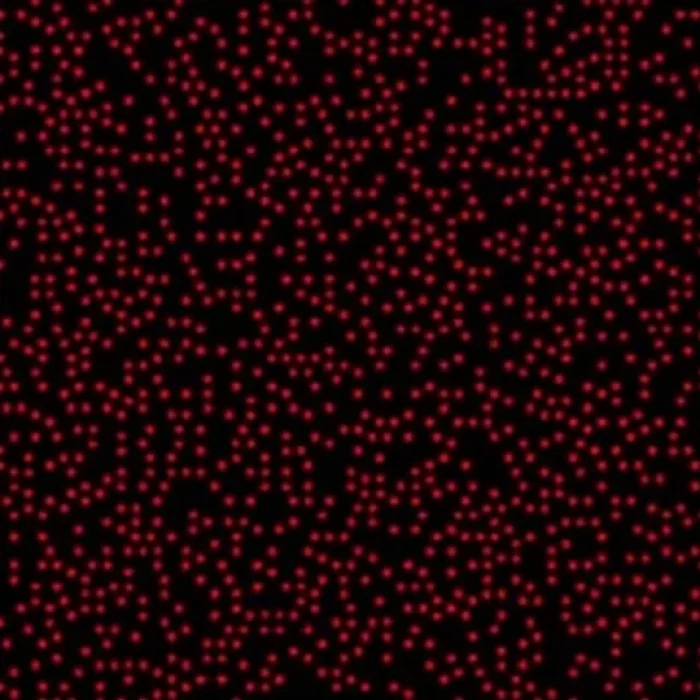
Argonne National Lab
Design of imaging lens for High-Energy X-ray Microscope to non-destructively study material stress in areas from 1cm square to atomic level.
2009
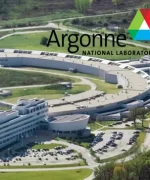
CellScope (sold to J&J)
Design of imaging lens and illumination optics for otoscope mounted on a smartphone, first commercial medical device based on smartphone
2012
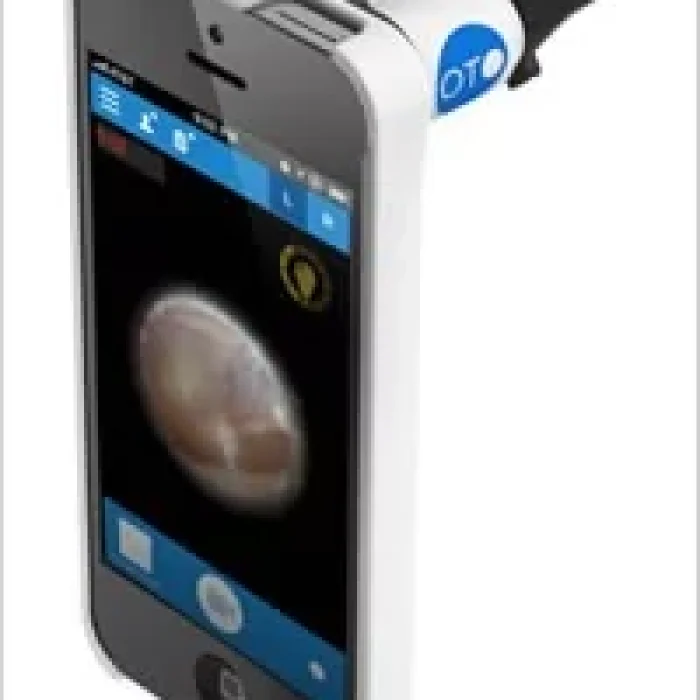
Our Project Process
How it works?
Our project review process is designed to ensure that every aspect of your project meets the highest standards of quality, precision, and performance. Here’s how we conduct our thorough project review:
How we work with clients:
From concept validation to analysis to design and production, every optical engineering project is different and based on your specific application area.
But these are typical stages of a project program:
Project Review: Discuss your project requirements, goals, and specifications to establish a clear foundation.
Concept Development: Paper design, simulations, and block diagrams for optics, mechanics, and electronics are created.
Design: Comprehensive design services, including optical, electronics, embedded software, mechanical design, and manufacturing documentation.
Prototyping: Prototype manufacturing and assembly are performed to create initial versions of the product for testing and evaluation.
Testing: Lens and subsystem testing, system debugging, experimental measurements, and prototype delivery..
Production Support: Developing quality control processes and delivering the system at volume.
Project Review: Discuss your project requirements, goals, and specifications to establish a clear foundation.
Concept Development: Paper design, simulations, and block diagrams for optics, mechanics, and electronics are created.
Design: Comprehensive design services, including optical, electronics, embedded software, mechanical design, and manufacturing documentation.
Prototyping: Prototype manufacturing and assembly are performed to create initial versions of the product for testing and evaluation.
Testing: Lens and subsystem testing, system debugging, experimental measurements, and prototype delivery..
Production Support: Developing quality control processes and delivering the system at volume.
Customer Feedback
“I have a background in optics, but lens design has always been a mystery to me. Your explanations helped me to decipher it.
Again, I am grateful for your expertise. It is a blessing for me to work with you and OFH.”
University of Alabama Birmingham
Development of ophthalmology research tool
“We really enjoyed working with you guys and your professionalism is admirable. We shall also revert back once we have the commercialization process sorted. “
Agrismart Engineering
Grow Light Design
“I want to take this opportunity to comment how impressed I am with OFH. Your team is moving so fast, I feel like a turtle. Also, it’s been noted that every time we ask something, our question is promptly answered and your team often brings up aspects that we haven’t considered.”
MTI Instruments
Industrial Distance Measurement Product
Experience and Innovation in Optical Engineering
With over 20 years of work history in optics, mechanics, and opto-electronics, we’ve developed more than 800 unique optical systems, proving that if it uses light, we’ve worked on it.
20+
Years of work history in optics, mechanics and opto eletronics
800+
Unique Optical Systems
Infinite
Variations if it uses light, we’ve worked on it
20+
Years of work history in optics, mechanics and opto eletronics
800+
Unique Optical Systems
Infinite
Variations if it uses light we’ve worked on it
Get in Touch
Every project begins with a conversation and review of your needs.
Start that process today!
Call Us
To discuss your project
781-583-7810
Email Us
We are ready to assist